Oxidative stress
Exploring non-coding RNAs (ncRNAs) in a piglet model of perinatal asphyxia
Perinatal- or birth asphyxia is caused by insufficient oxygen dispersion to the fetus and/or neonate at the time of birth resulting in over 680,000 deaths of newborns every year. The combination of hypoxia (insufficient oxygen supply), metabolic acidosis (increased acid levels), and ischemia (reduced blood perfusion) harms the vital organs of the neonates, including the heart, lungs, kidneys, and essentially the brain. A number of clinical and experimental animal studies indicate that perinatal asphyxia provokes the generation of reactive oxygen species (ROS) and the formation of oxidative stress. Reliable and early diagnostic markers for the severity and overall outcome of perinatal asphyxia could substantially improve intervention strategies and treatment.
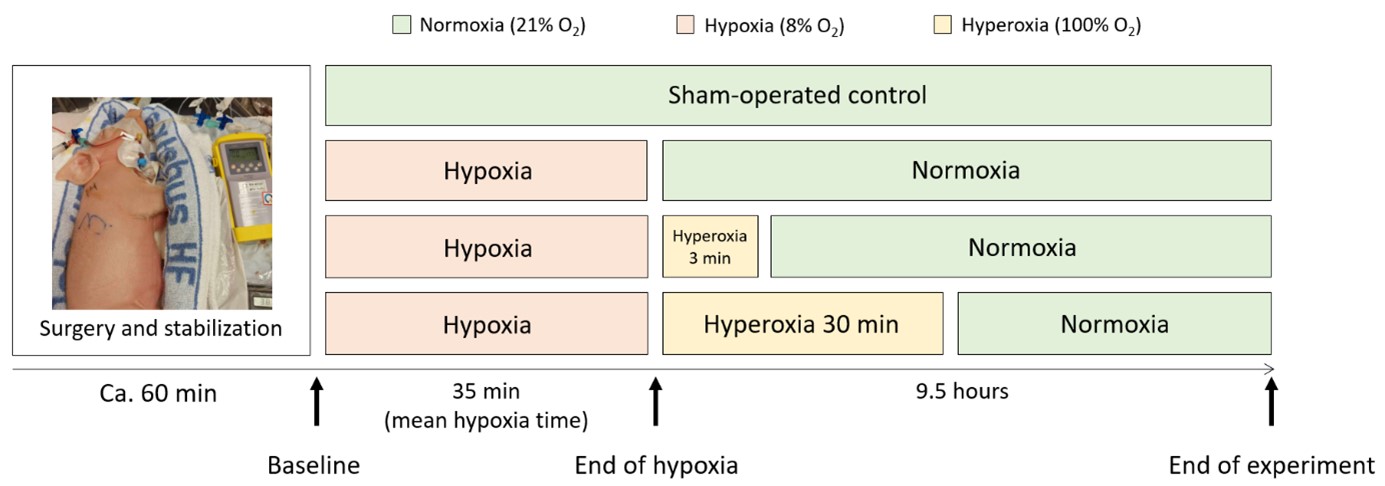
(From: Grebstad Tune et al., https://doi.org/10.3390/biology12040549; © 2023 by the authors).
It has been determined that around 75% of the entire human genome is transcribed; however, only 3% contribute to protein-coding genes. Non-coding RNAs are classified into two major groups, represented by short RNAs (less than 200 bp in length, including miRNAs) and by long non-coding RNAs (lncRNAs, represented with sequence lengths of 200 bp and above). In the last decade, it has become more and more evident that ncRNAs are involved in the regulation of many complex cellular and physiological processes and diseases, including oxidative stress, cellular senescence, aging and cancer. Examples of lncRNAs involved in DNA damage response and oxidative stress are H19, ANRIL, and MALAT1. Important target gene for these potential regulatory lncRNAs include VEGFA, BDNF, and HIF1alpha. We have presented one of the first studies to estimate sequential changes of ncRNAs in blood and tissue using a piglet model of perinatal asphyxia.
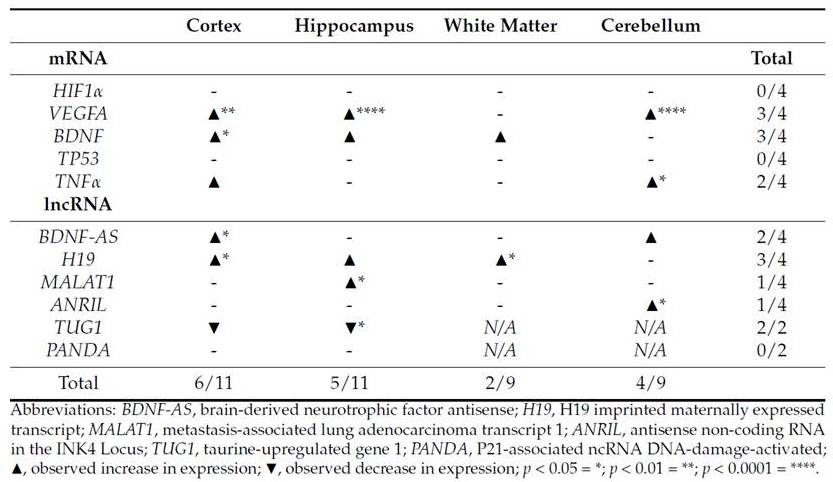
(From: Grebstad Tune et al., https://doi.org/10.3390/biology12040549; © 2023 by the authors).
This project resulted in the following publications:
Tune BG, Melheim M, Åsegg-Atneosen M, Dotinga B, Saugstad OD, Solberg R, and Baumbusch LO (2023) Long non-coding RNAs in hypoxia and oxidative stress: novel insights investigating a piglet model of perinatal asphyxia. Biology 12(4):549. doi.org/10.3390/biology12040549.
Garberg HT, Huun MU, Baumbusch LO, Åsseg-Atneosen M, Solberg R, and Saugstad OD (2017) Temporal profile of circulating microRNA after global hypoxia-ischemia in newborn piglets. Neonatology 111(2):133. doi.org/10.1159/000449032.
Investigating cell-free DNA (cfDNA) using a piglet model of perinatal asphyxia
An increase in cell free DNA (cfDNA) in plasma has been reported for a range of different diseases and conditions, including cancer, trauma, stroke, sepsis, heavy exercise and prematurity. It was first discovered in plasma but it has also been found in other body fluids, including cerebrospinal fluid and urine. CfDNA is usually composed of short DNA fragments of 150 – 250 base pairs (bp) in length. It has been hypothesized that an increase in cfDNA is a stress response to processes like cellular breakdown, reparation mechanisms (necrosis, apoptosis and autophagy) and tumor turn-over. Many different extraction and quantification methods have been presented for the assessment of cfDNA; however, a golden standard is still missing - in particular for non-human samples. We have investigated different methods for the extraction and quantification of cfDNA from different body fluids to investigate changes in cfDNA concentrations in a piglets model of neonatal hypoxia-reoxygenation.
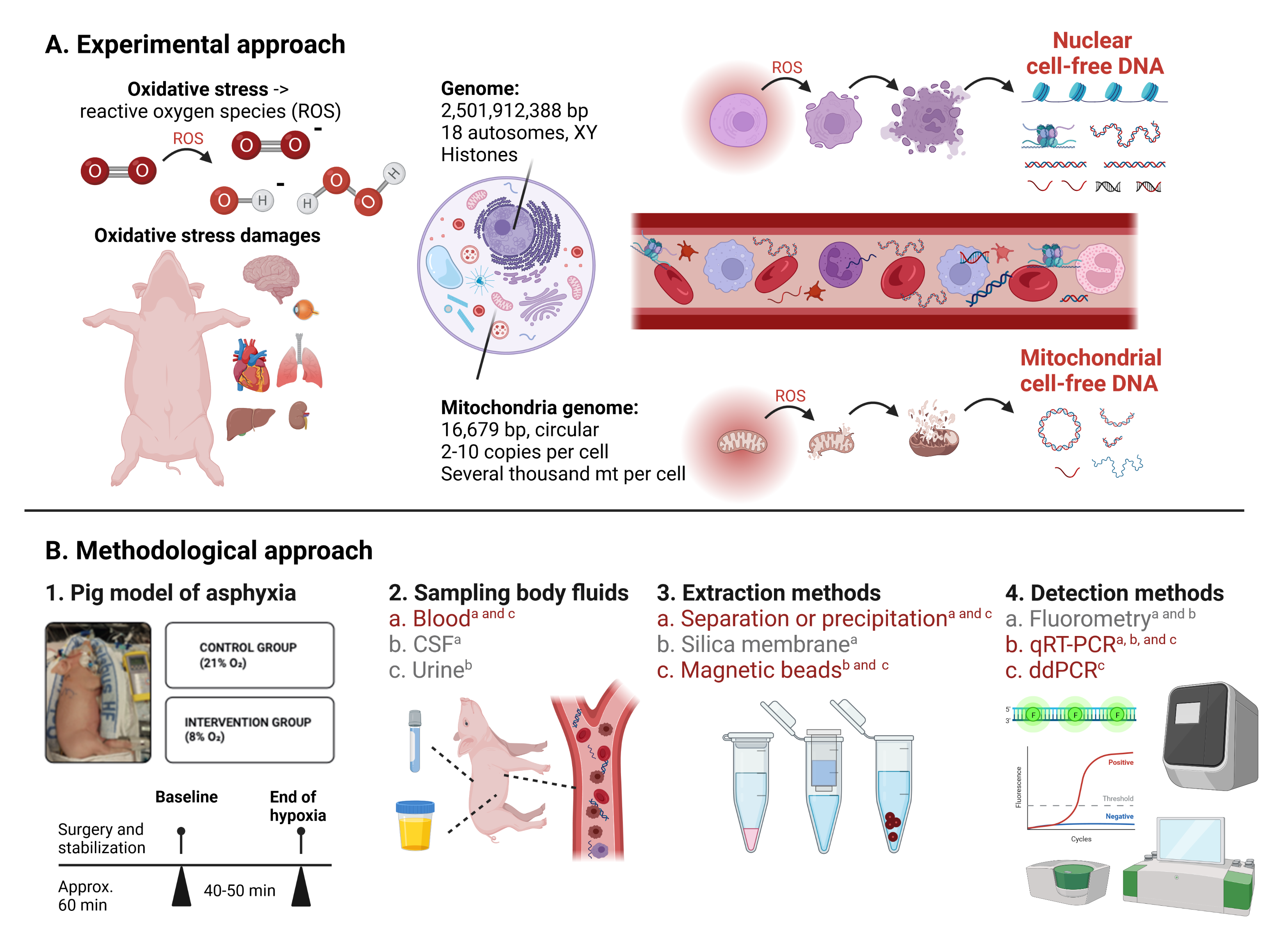
(From: Bitenc M. et al., https://doi.org/10.1007/s11033-022-08135-0; ©The Author(s) 2022).
This project resulted in the following publications:
Bitenc M, Tune BG, Melheim M, Åsegg-Atneosen M, Lai X, Rajar P, Solberg R, and Baumbusch LO (2022) Assessing nuclear versus mitochondrial cell-free DNA (cfDNA) by qRT-PCR and droplet digital PCR using a piglet model of perinatal asphyxia. Molecular Biology Reports 50(2):1533. doi.org/10.1007/s11033-022-08135-0.
Rajar P, Åsegg-Atneosen M, Saugstad OD, Solberg R, and Baumbusch LO (2019) Quantifiction of circulating cell-free DNA (cfDNA) in urine using a piglet model of asphyxia. PLoS One 14(12):e0227066. doi.org/10.1371/journal.pone.0227066.
Manueldas S, Benterud T, Rueegg RC, Garberg HT, Huun MU, Pankratov L, Åsegg-Atneosen M, Solberg R, Escobar J, Saugstad OD, and Baumbusch LO (2018) The temporal pattern of circulating cell-free DNA (cfDNA) in a newborn pig model of asphyxia damage. PLoS One 13(11):e0206601. doi.org/10.1371/journal.pone.0206601.
Novel biomarkers for neonatal asphyxia damage
Neonatal asphyxia is a severe medical condition resulting from oxygen deficiency (hypoxia) in newborn infants causing worldwide approximately 750.000 casualties per year. Infants surviving neonatal asphyxia frequently suffer from neurological complications, including cerebral palsy. Intervention strategies are limited, and there is still a lack of reliable diagnostic biomarkers for the prediction of the severity and outcome after neonatal asphyxia. Together with our collaboration partners, we examined genomic, transcriptomic, proteomic, and epigenomic changes after hyperoxia using piglet and murin models as well as pretermn patient samples.
This project resulted in the following publications:
Zasada M, Madetko-Talowska A, Revhaug C, Rognlien AGW, Baumbusch LO, Książek T, Szewczyk K, Grabowska A, Bik-Multanowski M, Pietrzyk JJ, Kwinta P, and Saugstad OD (2020) Transcriptome analysis reveals dysfunction of genes involved in oxidative phosphorylation in a murine model of retinopathy of prematurity. Pediatric Research 88(3):391. doi.org/10.1038/s41390-020-0793-x.
Revhaug C, Zasada M, Rognlien AGW, Günther C-C, Grabowska A, Madetko-Talowska A, Szewczyk K, Książek T, Bik-Multanowski M, Kwinta P, Pietrzyk JJ, Baumbusch LO, and Saugstad OD (2020) Pulmonary vascular disease is evident in gene regulation of experimental bronchopulmonary dysplasia. The Journal of Maternal-Fetal & Neonatal Medicine 33(12):2122. doi.org/10.1080/14767058.2018.1541081.
Zasada M, Madetko-Talowska A, Revhaug C, Rognlien AGW, Baumbusch LO, Książek T, Szewczyk K, Grabowska A, Bik-Multanowski M, Pietrzyk JJ, Kwinta P, and Saugstad OD (2019) Short- and long term impact of hypoxia on the blood and retinal cells' transcriptome in a murine mordel of oxygen induced retinopath. Pediatric Research 87(3):485. doi.org/10.1038/s41390-019-0598-y.
Zasada M, Suski M, Bokiniec R, Szwarc-Duma M, Borszewska-Kornacka MK, Madej J, Bujak-Giżycka B, Madetko-Talowska A, Revhaug C, Baumbusch LO, Saugstad OD, Pietrzyk JJ, and Kwinta P (2019) Comparative two time-point proteome analysis of the plasma from preterm infants with and without bronchopulmonary dysplasia. Italien Journal of Pediatrics 45(1):112. doi.org/10.1186/s13052-019-0676-0.
Revhaug C, Bik-Multanowski M, Zasada M, Rognlien AGW, Günther C-C, Książek T, Madetko-Talowska A, Szewczyk K, Grabowska A, Kwinta P, Pietrzyk JJ, Baumbusch LO, and Saugstad OD (2019) Immune system regulation affected by a murine experimental model of bronchopulmonary dysplasia: genomic and epigenetic findings. Neonatology 116(3):269. doi.org/10.1159/000501461.
Zasada M, Suski M, Bokiniec R, Szwarc-Duma M, Borszewska-Kornacka MK, Madej J, Bujak-Giżycka B, Madetko-Talowska A, Revhaug C, Baumbusch LO, Saugstad OD, Pietrzyk JJ, and Kwinta P (2018) An iTRAQ-based quantitative proteomic analysis of plasma proteins in preterm newborns with retinopathy of prematurity. Investigative Ophthalmology & Visual Science 59(13):5312. doi.org/10.1167/iovs.18-24914.
Suski M, Bokiniec R, Szwarc-Duma M, Madej J, Bujak-Giżycka B, Borszewska-Kornacka BK, Książek T, Grabowska A, Revhaug C, Baumbusch LO, Saugstad OD, Pietrzyk JJ, and Kwinta P (2018) Plasma proteome changes in cord blood samples from preterm infants. Journal of Perinatology 38(9):1182. doi.org/10.1038/s41372-018-0150-7.
Suski M, Bokiniec R, Szwarc-Duma M, Madej J, Bujak-Giżycka B, Kwinta P, Borszewska-Kornacka BK, Grabowska A, Revhaug C, Baumbusch LO, Saugstad OD, and Pietrzyk JJ (2018) Prospective plasma proteome changes in preterm infants with different gestation age. Pediatric Research 84(1):104. doi.org/10.1038/s41390-018-0003-2.
Benterud T, Manueldas S, Rivera S, Henckel E, Løberg EM, Norgren S, Baumbusch LO, Solberg R, and Saugstad OD (2017) N-Acetylcysteine amide (NACA) have potential neuroprotective effects on the cerebellum after perinatal asphyxia. Disease Markers 2018:5046372. doi.org/10.1155/2018/5046372.
Kwinta P, Bokiniec R, Bik-Multanowski M, Gunther CC, Grabowska A, Książek T, Madetko-Talowska A, Szewczyk K, Szwarc-Duma M, Borszewska-Kornacka MK, Baumbusch LO, Revhaug C, Saugstad OD, and Pietrzyk JJ (2017) Comparison of whole genome expression profile between preterm and full-term newborns. Ginekologia Polska 88(8):434. doi.org/10.5603/GP.a2017.0080.
Benterud T, Ystgaard MB, Manueldas S, Pankratov L, Alfaro-Cervello C, Florholmen G, Ahmed MS, Sandvik L, Norgren S, Bjørås M, Baumbusch LO, Solberg R, and Saugstad OD (2017) N-Acetylcysteine amide exerts possible neuroprotective effects in newborn pigs after perinatal asphyxia. Neonatology 111(1):12 doi.org/10.1159/000447255.
Benterud T, Pankratov L, Solberg R, Bolstad N, Skinningsrud A, Baumbusch L, Sandvik L, and Saugstad OD (2015) Perinatal asphyxia may influence the level of beta-amyloid (1-42) in cerebrospinal fluid: an experimental study on newborn pigs. PLoS ONE 10(10):e0140966. doi.org/10.1371/journal.pone.0140966.