Welcome to Lars Eide's group Mitogroup
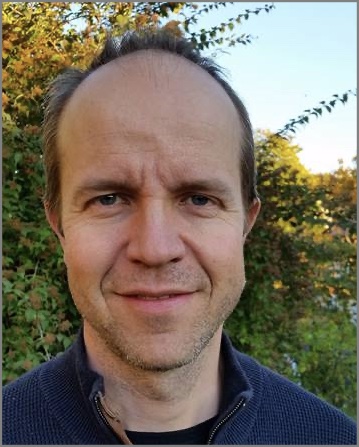
Group leader
Nuclear and mitochondrial genomes (nDNA and mtDNA, respectively) are subjected to dynamic modifications. There is a crosstalk between mitochondrial metabolism and mtDNA modifications.
Our aim is to understand how modifications on mtDNA influence/regulate mitochondrial metabolism.
Relevant diseases are:
- Maple Syrup Urine Disease (MSUD); a rare, inborn disease caused by deficient degradation of branched chain amino acids. This degradation is rate-limited by a large branched-chain keto acid dehydrogenase complex, whose core subunit is also a constituent of the “mitochondrial chromatin”.
- Pancreatic Ductal adenocarcinoma (PDAC) has reprogrammed energy metabolism towards branched-chain amino acid metabolism.
- Huntington’s disease (HD) is a neurodegenerative disorder, with strong association to mitochondrial dysfunction. We have found that nuclear DNA integrity is a peripheral biomarker that correlates with disease progression, and speculate that individual mitochondrial capacity dictates age of onset.
- Branched-chain amino acids are precursors for branched-chain fatty acids; with unknown function but present from gestation and throughout life, and positively associated with glucose homeostasis.
Various sources of oxidative stress can damage cellular molecules. The mitochondria host the machinery for aerobic convertion of energy from food into the cellular energetic currency ATP. Electrons enter the electron transport chain at distinct entry points. NADH from carbohydrate or protein catabolism, or beta-oxidation of fatty acids, FADH2 fromsuccinate dehydrogenase, an citric acid cycle intermediate complex and identified as complex II, whereas other proteins in the mitochondrial inner membrane transfer electrons to the lipid-soluble electron carrier: ubiquinone. Ubiquinone-carried electrons are translocated in the two-dimensional mitochondrial membrane to complex III, which delivers electrons to the water-soluble cytochrome C. Electrons from complex III finally reduce oxygen to water in complex IV. The second important feature of respiratory complexes, is the inherent property to channel protons across the mitochondrial membrane. In complex I, III and IV, this translocation is directed outward and establishes an transmembrane potential, which can be used for different energy requiring processes, such as transport, maturation of iron sulfur, and production of ATP from ADP ( in complex V).
In parallell with this process, mitochondria need to replicate their DNA (mtDNA). Because of the vicinity to the ETC, it has been argued that mtDNA is damaged more frequently from ROS than nuclear DNA. There are defined mtDNA repair systems, which prevent accumulation of oxidized lesions. The base excision repair pathway appears to be the most important in mitochondria, and we are using mitochondria from mtDNA repair deficient animals (such as the ogg1-/- mouse) in our experiments.
Mitochondrial maturation during neural cell differentiation
Neural stem cell differentiation activates aerobic metabolism with the concerted risk of introducing mtDNA damage because of increased side generation of reactive oxygen species. As a major defence, neural stem cells are equipped with robust mtDNA repair capacity towards oxidized lesions.
As an initial step to unravel the impact of mtDNA repair on functional differentiation, we analyzed mitochondrial maturation in neural stem cells devoid of OGG1, the major repair activity for removal of oxidized purines in the mtDNA. The results were reported in Stem Cells in 2010.